Turker Topcu
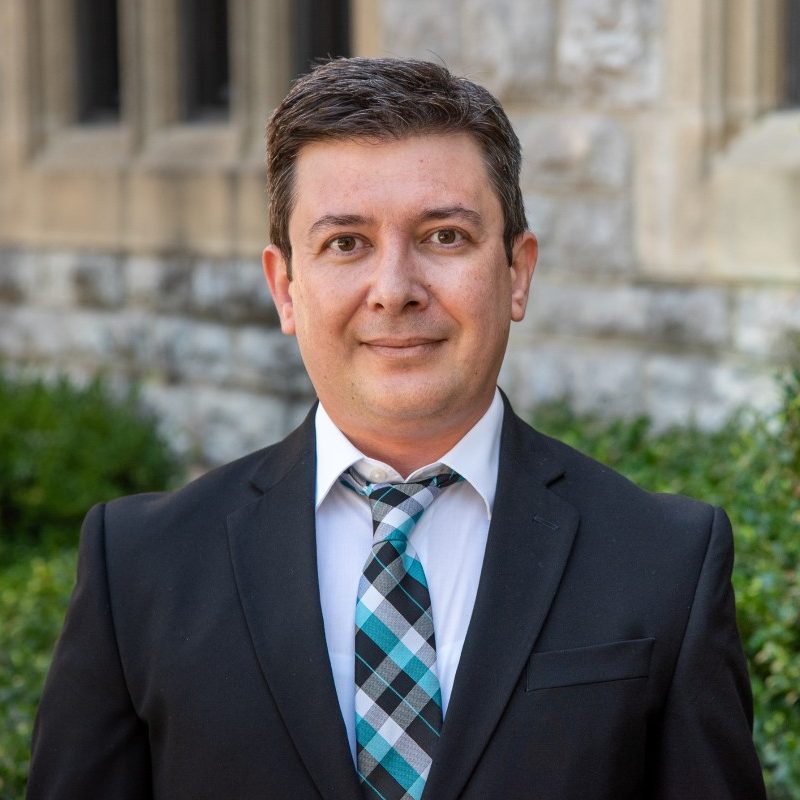
460 McBryde Hall, Virginia Tech
225 Stanger Street
Blacksburg, VA 24061-1026
Dr. Topcu works in the field of computational science. His research involves developing algorithms and codes to solve partial and ordinary differential equations to simulate quantum dynamical systems. Dr. Topcu's research is interdisciplinary and is currently active on two fronts: (1) Physical implementations of Quantum Information Processing (QIP) with neutral atoms, and (2) time-dependent simulations of atomic processes in the strong-field regime. The latter is directed towards exploiting emerging mechanisms to realize ultra-short light pulse generation to probe dynamical processes inside atoms and molecules in real-time. On the QIP end, he is focused on efficient quantum computing and entanglement schemes that utilize optically trapped cold Rydberg atoms.
1. Quantum computing with neutral atoms
Recent advances in precision time-keeping and quantum information science heavily rely on cold atoms trapped in optical lattices. For example, long-range interactions between neutral Rydberg atoms have emerged as a potential means for implementing quantum logic gates where conditional logic is mediated via the Rydberg blockade mechanism. For such gate operations to have high fidelity, several decoherence sources must be overcome. The experiments utilizing the Rydberg blockade require trapping both the ground state atoms and the Rydberg atom simultaneously. Since a Rydberg electron is weakly bound, the common wisdom has been that it behaves almost like a free electron and can only be trapped at an intensity minimum of an optical lattice. Our research has demonstrated that this view was not correct and showed that Rydberg atoms could be trapped at both intensity minima and maxima. We then devised a magic trapping scheme to exploit this for reducing both heating and dephasing in QIP experiments, eliminating an important source of error in quantum gate operations.
My current research in this area aims to further improve fidelity in QIP experiments. For example, creating an entangled set of spatially separated atomic clocks is directly relevant to Quantum Information Processing as such a clock network can also be utilized as a distributed platform for quantum computing by repurposing the clock states as qubit states. Besides its practical applications, entangling remote ensembles is also a topic of fundamental interest in atomic, molecular and optical (AMO) physics.
My work on magic trapping divalent atoms in optical lattices has become particularly helpful in designing such an entangling protocol that minimizes errors. Time-dependent simulations from first principles to design and evaluate quantum logic gates that can exploit non-perturbative atomic processes have been mostly absent. Such simulations can evaluate processes such as multi-photon excitations and long-range interactions for use in QIP protocols. For example, one of the limiting factors in QIP experiments is the ionization of Rydberg states by the trapping optical fields: ionization reduces the lifetime of the Rydberg state. As a result, estimates for the ionization lifetimes of Rydberg states go into the error estimation of every quantum gate scheme exploiting the Rydberg blockade mechanism.
Current estimates of these ionization lifetimes assume that the dipole approximation is valid for describing the interaction of the Rydberg atom with the external laser field. Computational approaches for evaluating gate protocols are particularly useful in these problems. For instance, computational studies can help develop a better understanding of the ionization dynamics of Rydberg atoms in optical lattices by taking the intensity landscape into account, and by going beyond the dipole approximation.
2. High harmonic generation: atoms coupled to nano-structures
A variety of rich and interesting phenomena emerge when ground state atoms and molecules interact with intense laser pulses, such as strong field ionization (SFI) and high-order harmonic generation (HHG). The wide range of photon frequencies generated in HHG can be used to compose ultra-short (attosecond) pulses, which opens the door for controlling ultrafast atomic and molecular processes at the natural time scale of the electron motion. Some of the applications include resolving electronic orbital structures with a high degree of spatial and temporal resolution and controlling and imaging the electron dynamics in molecules.
In a recent project, we investigated HHG an atom confined inside a C60 molecule. We find that the intensity of the generated harmonics can be several orders of magnitude larger when compared with the spectrum from a free H atom. This enhancement can be used to increase the intensity of attosecond pulses that can be composed from HHG spectra, making it possible to probe atoms more efficiently at the attosecond time scale.
Future extensions of this project will investigate compounding different enhancement mechanisms resulting from coupling one- and two-electron atoms to other kinds of nanostructures. For example, we recently showed that coupling atoms to wedge-shaped nanostructures also enhances HHG intensity.